In this article, we will walk together step by step into one of the most fascinating worlds of quantum physics. Bring your curiosity and imagination and let’s get started!
What is physics?
Lets start from the basics, what is physics? Physics is the study of the behavior of nature, how things move, and what they’re made of. Quantum mechanics is the part of physics that delves into the behavior of the tiniest particles in the universe. Its strange nature has sparked debates among scientists and thinkers, challenging our classical understanding of reality with its probabilistic view. However, through rigorous experimentation and advanced theoretical developments, we now possess a more refined and sophisticated understanding of quantum mechanics, which continues to reshape our fundamental perceptions of the physical world.
In this article we will introduce the fundamental principles of quantum physics, drawing from renowned historical experiments. This historical approach will help you grasp why scientists were led to draw counterintuitive conclusions on the rules that our world follows. We aim to present these concepts in a manner that surpasses the sensationalism and the hype often linked with the field.
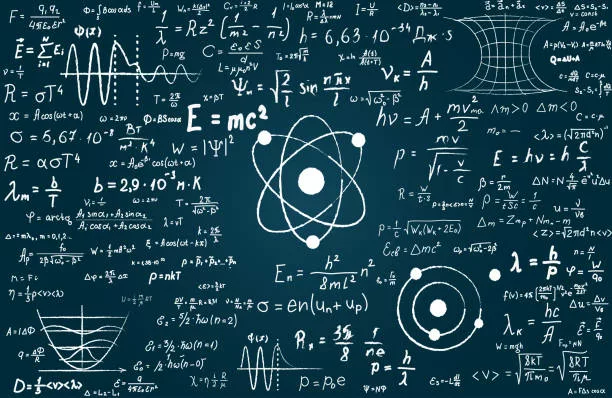
Is Light a wave or a particle ?
In classical physics (simply, this term refers to physics before quantum physics), objects are typically described either as particles or waves, depending on their characteristics and behavior. The question of whether light is a wave or a corpuscle was central to the foundation of quantum physics.
Particles are considered discrete entities with definite positions and speeds. Think of a ball rolling down a hill or a car moving along a road โ They follow deterministic paths, and they have properties, such as mass and velocity.
Waves such as sound waves ripples on the surface of the water, are characterized by their propagation through a medium. They have properties such as interference, diffraction, and superposition. When you throw two small stones into a pond, you can see patterns where the ripples overlap โ sometimes they make bigger waves (constructive interference), and sometimes they cancel each other out (destructive interference).
Should light be seen as a particle, or a wave?ย This question bothered scientists for centuries, and the debate was not easily settled.
In the 17th century Sir Isaac Newton proposed that light consists of particles, because he observed that light travels in straight lines and can cast sharp shadows. These observations led him to believe that light behaves more like a stream of particles rather than waves.
On the contrary, in the 1800s, experiments by scientists like Thomas Young and Augustin-Jean Fresnel provided strong evidence for the wave nature of light by showing the diffraction and interference of light which are wave properties. But waves require a medium like air or water to propagate. It is not the case for light, since it can travel through space, between the Sun and the Earth.
These questions were a unexplainable with classical physics driving the need for a new paradigm: quantum physics.
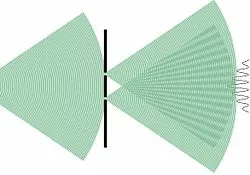
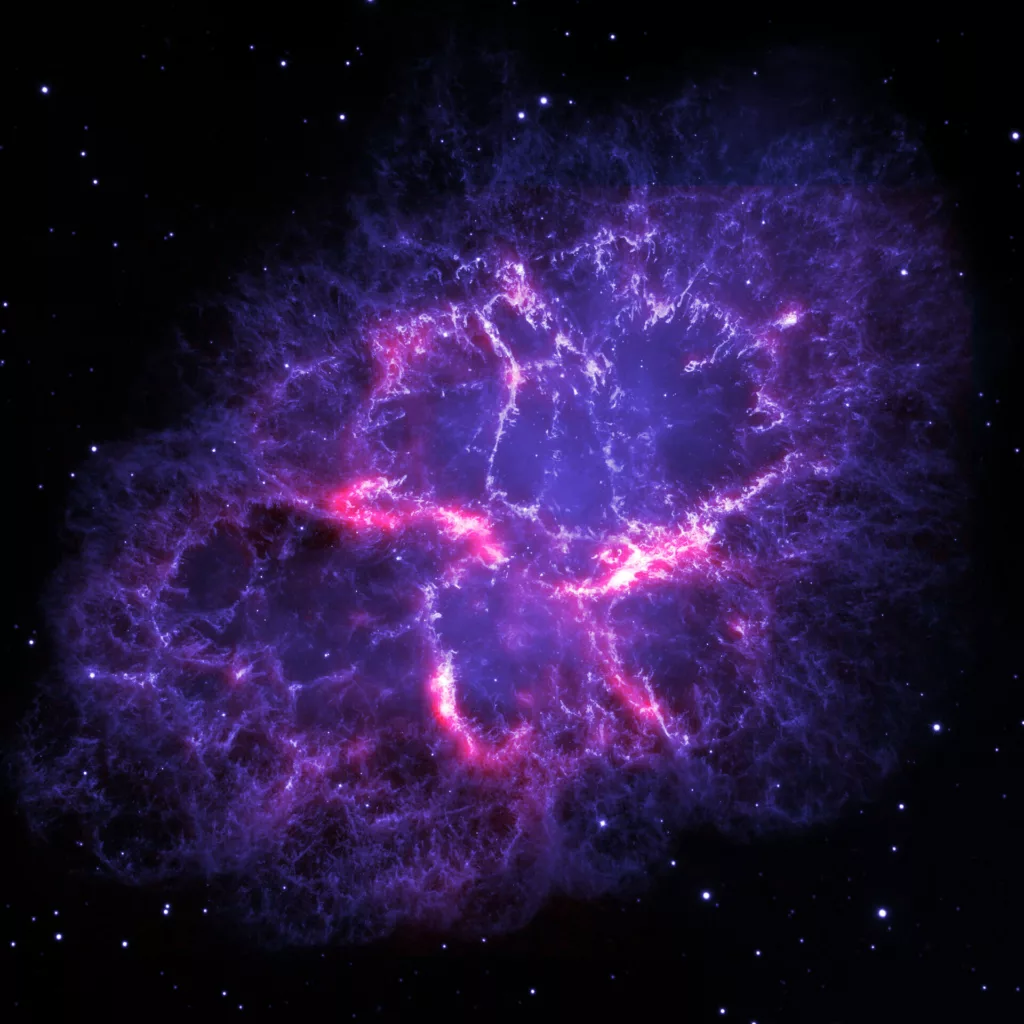
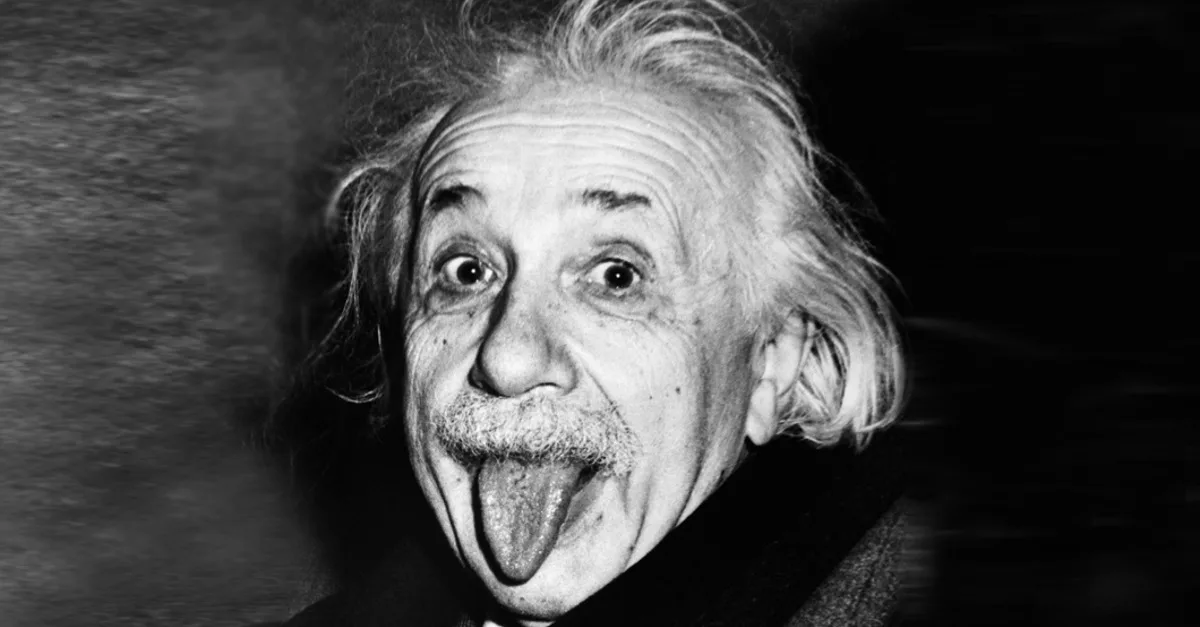
The notion of Quanta
The first component of quantum physics was introduced in 1900 when the physicist Max Planck noticed that energy is not emitted or absorbed continuously by matter but rather in discrete units or quanta.
In 1905, in an attempt to answer whether light is a wave or a particle Albert Einstein proposed the idea of light quanta – which has since then been regularly observed in experiments. Einsteinโs concept of light quanta exhibits behaviors belonging to the “wave” category such as interference as well as traits akin to “particle” behavior. Yet, it doesn’t squarely fit into either category. To make it more palpable, we can say that it’s both. This revolutionary idea laid the foundation for quantum physics.
The wave-particle duality
Twenty years later, scientists discovered that matter itself could exhibit wave-like behaviors, just like light. This understanding emerged by observing electron interference. This phenomenon is particularly striking in the double-slit experiment, which involves shooting individual electrons through two closely spaced slits onto a screen. Surprisingly, instead of forming two distinct bands as expected with particles, an interference pattern emerges, as if it was a wave. We recommend watching the following video produced by the YouTube channel ‘La Physique Autrement’ to better visualize this phenomenon.
After a lot of head-scratching, scientists finally sorted things out and found the concepts to explain it all. In the following sections of the article, we’re going to lay out these rules which tell us how matter and light behave in the small world.
Quantum state and superposition
Quantum states: In quantum mechanics, an object is described by a quantum state. It encapsulates all the information that can be known about it at a particular moment, including properties such as position, velocity, energy, etc.
Superposition: Superposition essentially describes the situation where a quantum system can exist in multiple states at once. For example, in the double-slit experiment, interference occurs because electrons can take multiple paths simultaneously due to superposition. This means that each electron has the ability to pass through both slits simultaneously, resulting in a wave-like interference pattern when they interact with each other.
Consequently, certain positions on the screen are never reached due to destructive interference. This is a concrete example of when matter, such as an electron, behaves as a wave. It must be noted that when a measurement process is applied, the quantum system collapses to a single state. The phenomenon is known as wave function collapse, and destroys the superposition.
Superposition lies at the heart of quantum technologies, exemplified by the concept of a quantum bit, or qubit, which diverges from the classical bit’s binary nature. While a classical bit can only be in the state of 0 or 1, a qubit can exist in a superposition of both states simultaneously. This remarkable attribute grants quantum computers unparalleled potential for tackling intricate problems
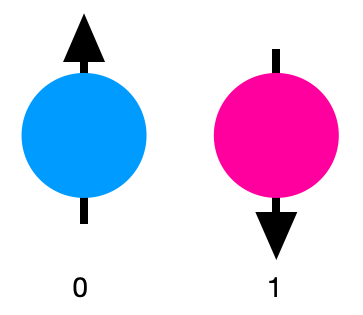
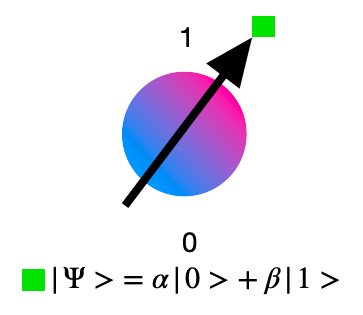
Entanglement
Imagine you have two magical coins, and you give one to your friend who lives far away. These coins are special because they’re entangled. Now, whenever you flip your coin and it shows heads, your friend’s coin instantly shows tails, and vice versa, no matter how far apart you are. This spooky connection is what scientists call “entanglement.”. So, if you check your coin and see heads, you know your friend’s coin must be tails, even if they’re on the other side of the world! It’s like they’re connected by an invisible string that lets them communicate instantly.
This strange behavior, where one coin’s state instantly affects the other’s, is what we call non-locality : the two coins are two bounded constituents of the same โentangled objectโ, and yet they are not necessarily located in the same place. It’s one of the most mind-boggling aspects of the quantum world, and yet it is achieved every day in quantum physics laboratories with atoms, or photons!
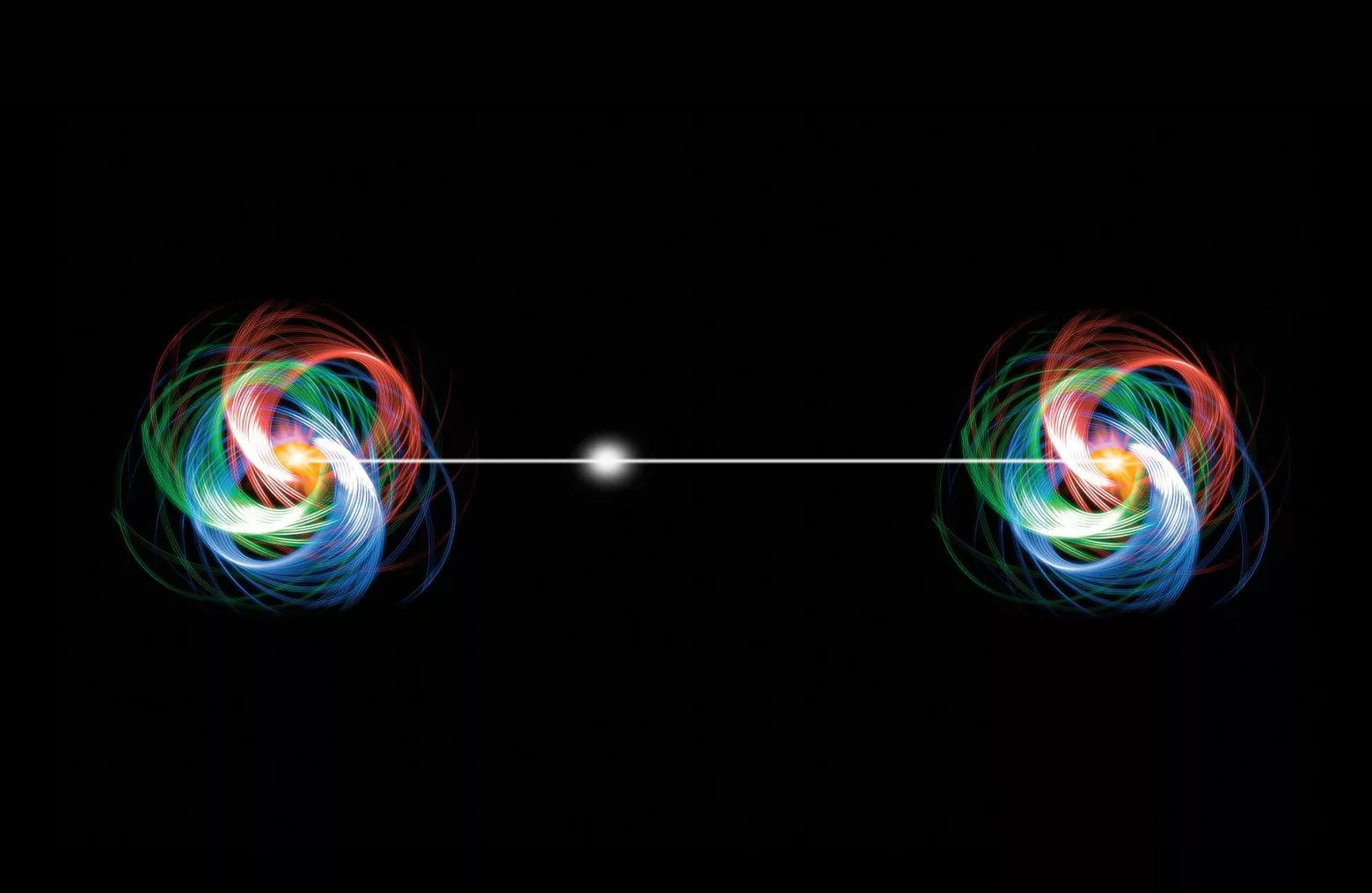
Schrรถdinger cat’s decoherence, quantum phenomena at large scales?
Decoherence is the process by which quantum systems lose their quantum properties such as superposition and entanglement. It is due to the interaction of the quantum particle with the environment, for example when you measure it, or look at it. We say the quantum state collapses and so we lose the quantum properties.
Decoherence is like lifting the veil on a magic trick. Instead of something mysterious: a quantum superposition of dead and alive states, we find a straightforward 50-50 chance of the cat being either dead or alive. Decoherence is the reason why we can’t see weird quantum stuff in everyday life, like Schrรถdinger’s cat being both dead and alive. Conversely, when an atom or an electron is isolated from its environment, decoherence is reduced and the quantum behavior is preserved. We can see in the double-slit experiment that the scientists have managed to reduce the decoherence as much as possible to make electrons interfere.
ย Decoherence is the strongest enemy of quantum technologies, as it stops us from conserving a quantum state for a long time. That’s why designing a long quantum algorithm is no easy feat.
You are now familiar with the core ideas of quantum physics, yet it is important to address how scientists agreed on these non trivial behaviors. The following section revolves around the debate between Einstein and Bohr on whether these behaviours are real. Over time, experiments and new theories have helped clarify this debate, which does not hold anymore.
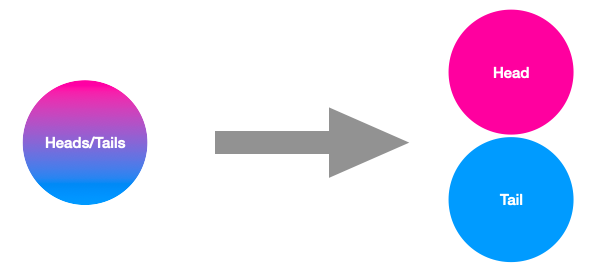
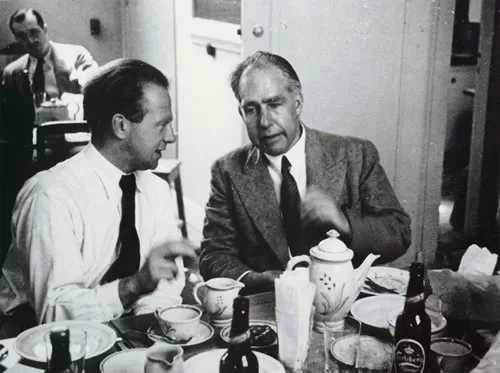
The Copenhagen Interpretation
The Copenhagen interpretation, pioneered by Niels Bohr, Werner Heisenberg, and Max Born, is one way of understanding the strange and fascinating world of quantum mechanics on a philosophical standpoint. It still holds paradoxes but it is the interpretation that is the most accepted today.
In everyday life, we’re used to thinking of things being in definite states. For example, a light switch is either on or off. But in the quantum world, things behave differently. According to the Copenhagen interpretation, at a very small scale, particles like electrons and photons don’t have definite properties until they are observed. Instead, they exist in a kind of fuzzy state of possibilities (superposition of multiple states), where different outcomes are possible with certain probabilities. It is only the observation of the object which will select a specific value of these properties.
A proof of entanglement
EPR paradox
Named after Einstein, Podolsky, and Rosen, the EPR paradox challenged the Copenhagen interpretation, arguing that quantum mechanics phenomena contradict Einsteinโs theory of relativity. In fact, the ultimate speed limit in the universe is the speed of light which is finite. However, Non-locality in quantum mechanics – i.e. quantum entanglement – implies faster-than-light communication according to Einsteinโs team. They concluded that quantum mechanics must be incomplete. Albert Einstein, focusing on this problem in his later years, tried to introduce hidden factors to avoid the implications of non-locality.
Bell’s Inequality
In 1964, physicist John Bell formulated an inequality that any classical system must respect, but not all quantum systems – according to Bohr’s interpretation. This solidified the paradigm shift initiated by the Copenhagen interpretation, as it showed that Einstein’s and Bohr’s description of quantum physics could lead to physically different behaviours.
French physicist Alain Aspect conducted experiments that ended the debate, showing that quantum mechanics does indeed violate Bellโs inequality. For his work in the 1980s, A. Aspect was awarded the Nobel Prize in Physics 2022.
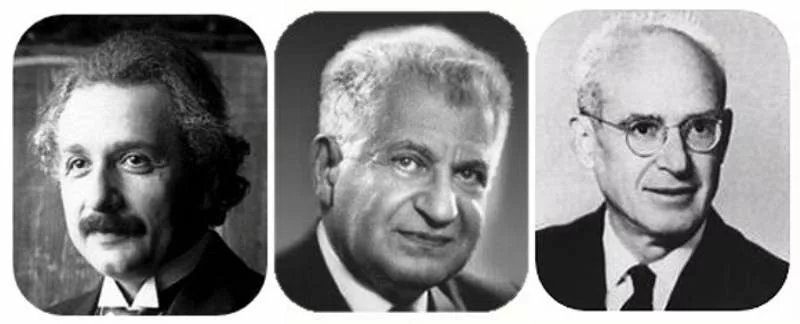
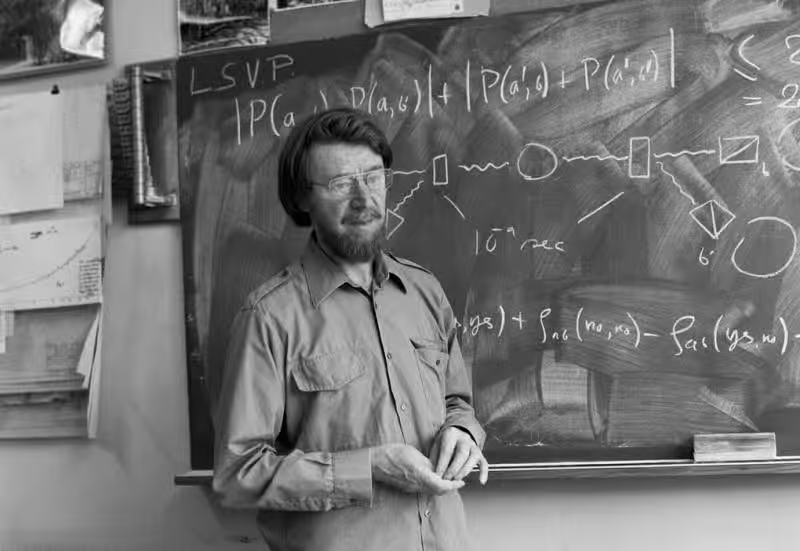
Conclusion
Dear reader, I trust you found this exploration of the foundations of quantum mechanics intriguing. You already know all the main phenomena that govern our world at small scales and can understand how quantum technologies work! Presently, we find ourselves amidst the second quantum revolution, marked by exciting developments and endeavors. Promising applications and projects abound, including advancements in quantum computing, quantum internet, quantum sensing, and quantum cryptography. The landscape of technology is evolving rapidly!